We are committed to sustainability
The following is an executive summary of LCA report reference ED18376101 which was critically reviewed by Ricardo in accordance with the requirements of UNI CEN ISO/TS 14071:2016 (ref. par. 4.3.3).
Methanol is an important and highly versatile chemical used to produce hundreds of every-day products. Examples of end-products that can be synthesised using methanol as an intermediate include but are not limited to; clothing materials such as synthetic fabrics and fibres, construction materials including adhesives, paint and plywood, derivative products such as acetic acid and formaldehyde and as a fuel. For construction materials, it is likely that the impact associated with methanol would be sequestered due to the long lifetimes of buildings and other construction products.
Furthermore, methanol can be a cleaner-burning alternative to conventional fuels and a potential enabler for decarbonisation. When methanol is used as a combustion fuel, or as feedstock to produce fuels like kerosene (SAF), gasoline or dimethyl ether (DME), the combustion emissions would be released to the environment. This means that the accumulated emissions in the value chain become very important to the overall decarbonisation potential of methanol-based fuels.
JM is committed to reducing the impact of its technology on the planet. As a leading provider of methanol technology, JM seeks to understand the relative impact of its methanol technologies. This is done by conducting a Life Cycle Assessment (LCA) study to measure and compare the environmental impact of JM methanol production flowsheets, which are licenced to customers for methanol production.
The study compares natural gas-based flowsheets of methanol technologies: BALANCE™, SWITCH™, PRECISION™, eMERALD™ CO2 to methanol process (e-methanol) and the biomass to methanol (biomethanol). The results are then compared to a steam methane reforming (SMR) benchmark. The data used for this study reflects the likely applications of JM methanol production flowsheets and is presented in the table below:
Methanol Technology |
Carbon feedstock |
Hydrogen feedstock |
Electricity source |
eMERALD (e-methanol) |
Point-source CO2: CCU from bioethanol production |
H2 electrolysis |
EU renewable energy mix |
Biomass to methanol (Biomethanol) |
Residual wood from primary forestry industry |
EU renewable energy mix |
|
BALANCE |
Natural gas (EU market average) |
EU grid mix |
|
PRECISION |
Natural gas (EU market average) |
EU grid mix |
|
SWITCH |
Natural gas (EU market average) |
EU grid mix |
The study is conducted following the iterative approach of the ISO14040:2006+A1:2020 and ISO 14044:2006 standard methodologies, and guidance from the ‘Together for Sustainability Product Carbon Footprint’ guidelines. The study is third party verified by Ricardo.
The scope of the study is cradle-to-gate and includes the temporary sequestration of CO2 into the final methanol product, where applicable. The default geography is Europe, and the functional unit of study is 1 MJ (Low Heating Value) of methanol.
The potential for decarbonisation offered by JM’s methanol technologies is assessed using global warming potential (GWP) as an impact metric. The International Panel on Climate Change (IPCC) provides two methods that quantify GWP at 100 years: IPCC 2021 GWP100 and IPCC 2021 GWP100 (incl. CO2 uptake). In the former method, IPCC 2021 GWP100, carbon dioxide uptake is implicitly included by excluding both CO2 uptake and emissions of biogenic CO2, under the assumption that uptake and emissions of biogenic CO2 have net zero impact. Biogenic methane emissions are explicitly accounted for in this method. The IPCC 2021 GWP100 (incl. CO2 uptake) method explicitly includes both CO2 uptake and biogenic CO2 emissions.
The study demonstrated that JM’s eMERALD (e-methanol) and biomethanol processes are credible routes to decarbonisation of methanol production versus JM’s natural gas-based processes and those of the SMR benchmark.
JM’s e-methanol and biomethanol processes have the potential to deliver between 35-39% reduction in global warming potential compared to the SMR benchmark using the IPCC GWP100 methodology, and the potential of up to 185% reduction for biomethanol and 143% for e-methanol versus the same SMR benchmark when using GWP100 (incl. CO2 uptake).
JM’s SWITCH methanol process has the potential to reduce GWP by 15% versus the SMR benchmark, compared to 1% potential reduction for BALANCE and PRECISION methanol processes. SWITCH has even further potential for reduction of global warming potential (up to 28%) if renewable electricity can be utilised.
The LCA results confirm that feedstocks are the largest contribution to the global warming potential of methanol production and its contribution is sensitive to the impact assessment methodology used. The chart below demonstrates the results from the cradle-to-gate impact assessment for GWP100 incl. CO2 uptake, both with and without the contribution of feedstocks.
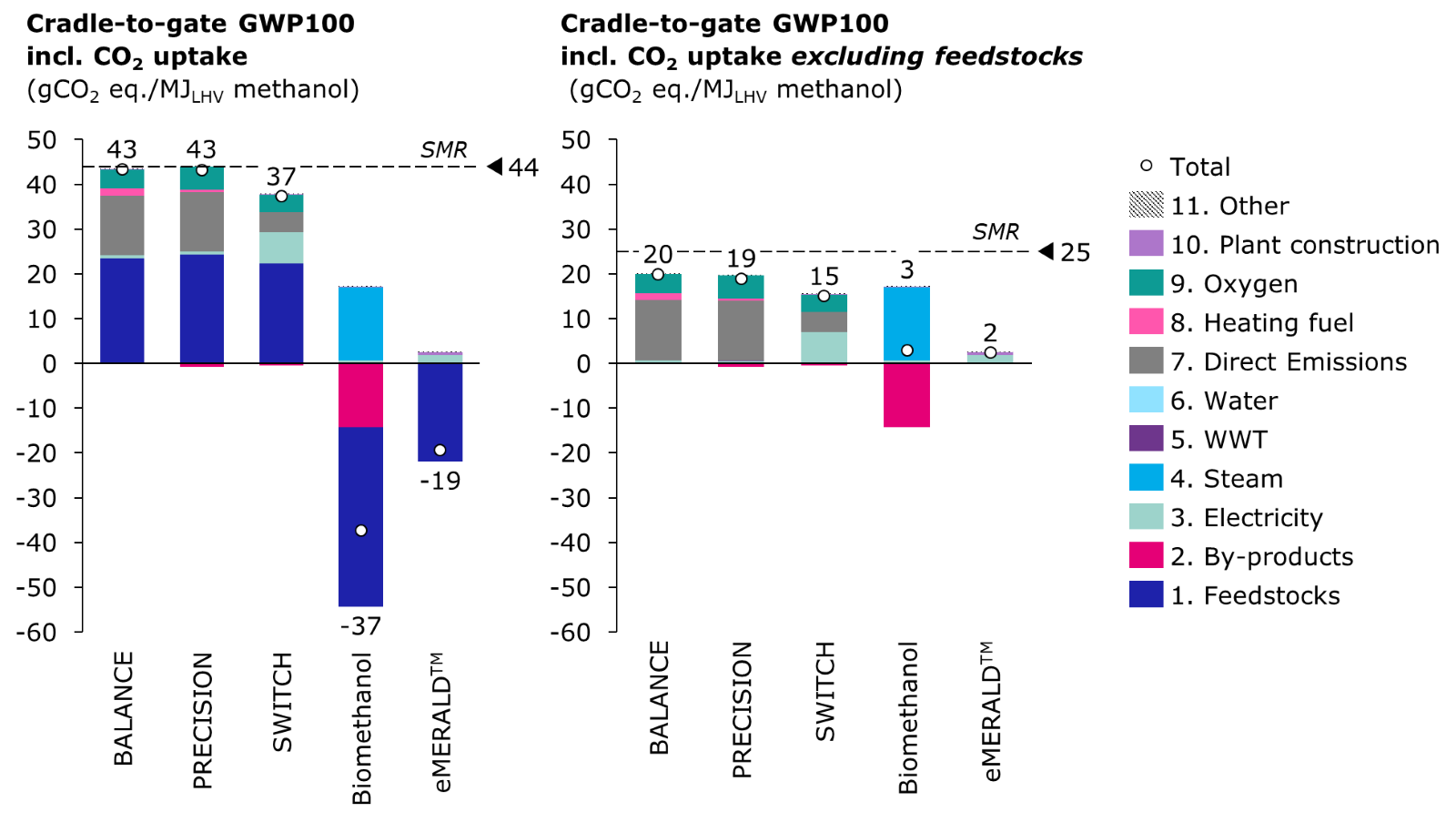
Figure A. Comparison of GWP100 incl. CO2 uptake and GWP100 (kg CO2 eq./MJ methanol) of five different methanol technologies, broken down by impact source. Standard steam methane reforming (SMR) methanol benchmark is included for reference based on LCI from Mahabir et al. (2022).
The net negative GWP results for biomethanol and e-methanol are predominantly attributed to the temporary sequestration of CO2. For e-methanol, in addition to temporary sequestration, the use of a renewable H2 from electrolysis also aids in decarbonisation. Biomethanol production was sensitive to the source of oxygen required for biomass feedstock gasification and the type of biomass feedstock, whilst e-methanol production is highly sensitive to the renewable electricity mix used for hydrogen electrolysis.
When excluding the feedstock and considering only the impacts directly associated with methanol production, e-methanol represents the lowest global warming potential, followed by biomethanol. Both biomethanol and e-methanol deliver lower global warming potentials than the natural gas production routes.
Other impact factors considered in the study were aligned to the EU Product Environmental Footprint guidelines, as well as JM’s environmental footprint methods, including water deprivation. AWARE water use within JM’s methanol technology flowsheets is less than 5% of the overall impact, as the largest water deprivation impact is due to feedstock production. For biomethanol this sensitivity is due to the source of oxygen required for biomass gasification, and for e-methanol it is the renewable electricity required to produce the hydrogen feed. For natural gas to methanol technologies, water deprivation impacts are dependent on the efficiency of extraction, which varies with geography.
Biomethanol and e-methanol technologies offered by JM were designed to enable the de-fossilisation of methanol production. Consequently, these renewable methanol technologies are advantageous when considering total GWP100 and fossil cumulative energy demand (CED) impact metrics. Other environmental impacts considered in this study highlight the need for further improvement in the feedstock supply chain for biomethanol and e-methanol. Key areas of focus should be renewable electricity and feedstock sourcing as well as electrolyser and gasification technology. Advances in these areas for biomethanol and e-methanol can make significant improvements in their wider environmental impact.
The following conclusions can be drawn from the impact assessment methods used in this study, including the global warming potential and AWARE discussed above on a cradle-to-gate basis:
-
The use of eMERALD (e-methanol) and biomethanol technologies present credible routes to decarbonising methanol production.
-
JM’s SWITCH methanol provides a 15% reduction in global warming potential compared to the SMR benchmark. This reduction can be increased to 28% if renewable electricity is available.
-
When assessing the technologies across the range of impact factors in the study, e-methanol and biomethanol have the lowest impacts for global warming potential and fossil cumulative energy demand. Significant improvements for the wider environmental impacts of e-methanol and biomethanol, however, can be made with careful consideration of:
-
renewable electricity and feedstock sourcing, and advances in electrolyser and gasification technology.
For more information, please get in touch with our methanol team.