Technical Brief
Nickel complexes for cross-coupling
Leveraging novel chemistries to enhance the cross-coupling toolbox
In recent years, the advancement of base metal catalysis, particularly nickel, in various industrial applications has received significant attention in scientific literature (1). A major benefit of homogeneous nickel catalysis is that it enables the use of novel chemistries and substrates in synthetic applications that can’t be achieved with stoichiometric reagents or other catalytic technologies. For example, nickel catalysis uniquely unlocks photoredox, energy transfer, and electrochemical methodologies to further enhance the synthetic toolbox available to both industrial and academic chemists.
In cross-coupling applications, homogeneous nickel-phosphine or nickel-bipyridyl catalyst systems have been shown to activate an orthogonal array of electrophilic substrates for coupling when compared to copper or palladium-based catalyst systems. Johnson Matthey (JM) is now offering a selection of catalysts and precursors to support these developing applications of nickel homogeneous catalysis.
In situ catalyst precursors
Ni(COD)2 (Ni-129)
Ni(COD)2 is a precursor for in situ catalyst generation that has been used in a wide variety of applications since its introduction in 1960. Due to its favourable solubility properties and high ligand lability, Ni(COD)2 has seen extensive use in the polymer industry as both a catalyst precursor and co-catalyst (2).
More recently, Ni(COD)2 has been shown to be an effective catalyst precursor for a variety of novel applications:
- Catellani reaction (3)
- Kumada sp3-sp3 couplings of isotopically labeled nucleophiles (4)
- Atroposelective C-H alkylation of benzimidazoles (5)
- Energy transfer catalysis for sp2-sp3 bond construction with novel TADF materials (6)
- Catalytic degradation of a BPA epoxy resin (7)
- Functionalization of phenol derivatives (8)
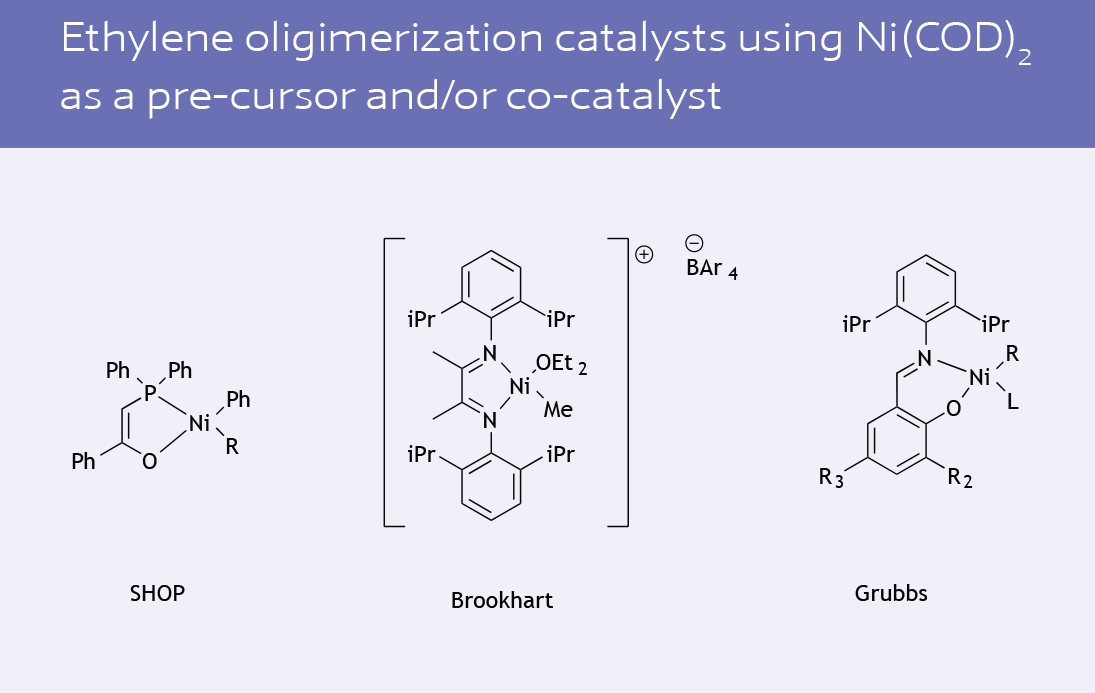
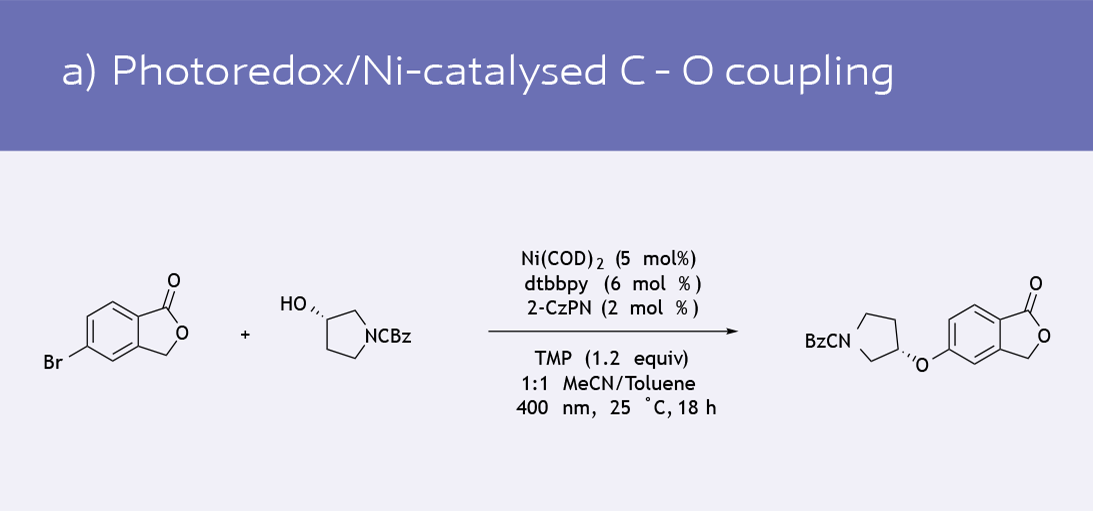
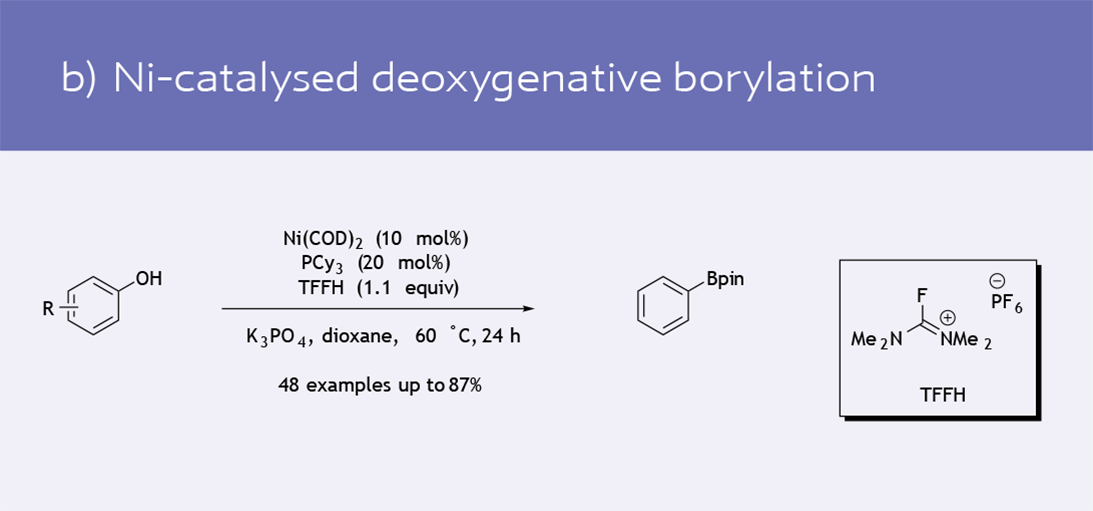
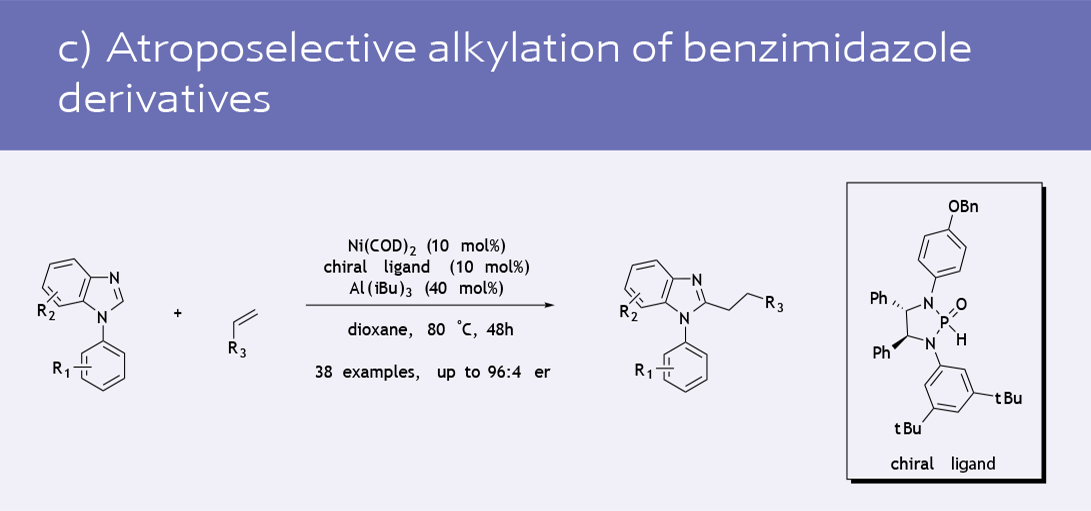
Selected recent catalytic transformations using Ni(COD)2 as the catalyst precursor
In situ catalyst precursor
Ni(COD)(DQ) (Ni-131)
While Ni(COD)2 is a versatile option, there are challenges in its use in industrial processes due to its air sensitivity. The development of a general, air-stable Ni(0) precursor has been highly sought after in recent years. Ni(COD)(DQ) is an air and shelf-stable precursor for in situ catalyst generation introduced by the Engle lab in collaboration with an industrial partner (9).
Since its introduction to the chemical industry, Ni-131 has seen various applications as a catalyst precursor:
- Carbon isotope exchange of aryl nitriles (10)
- Preparation of polythiophene materials (11)
- Cross coupling of sulfonamides with aryl bromides (12)
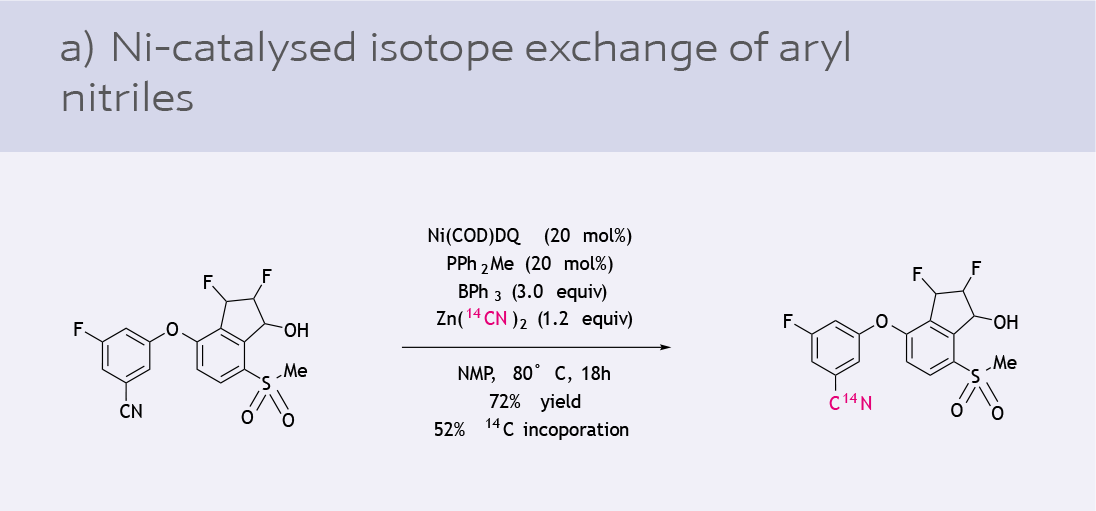
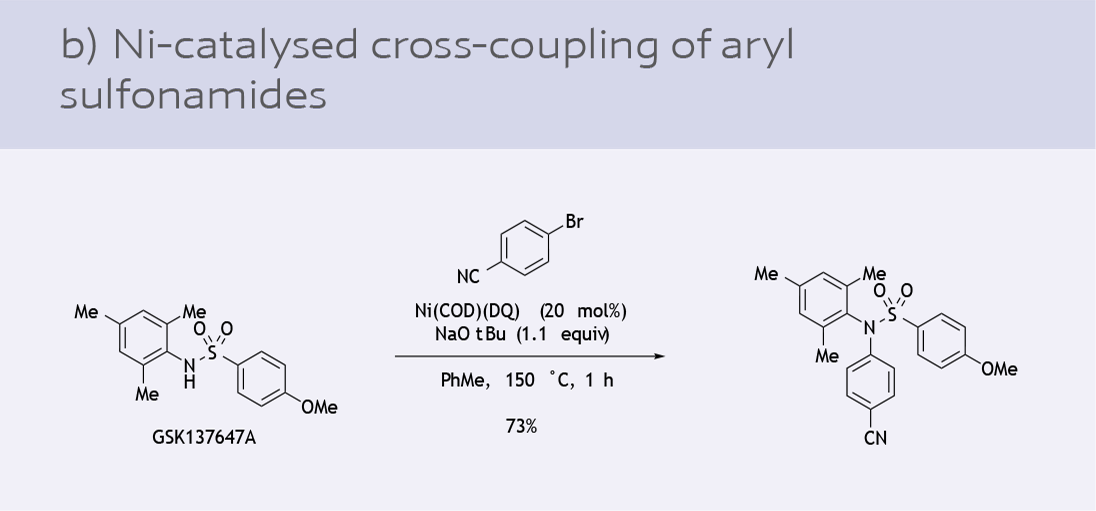
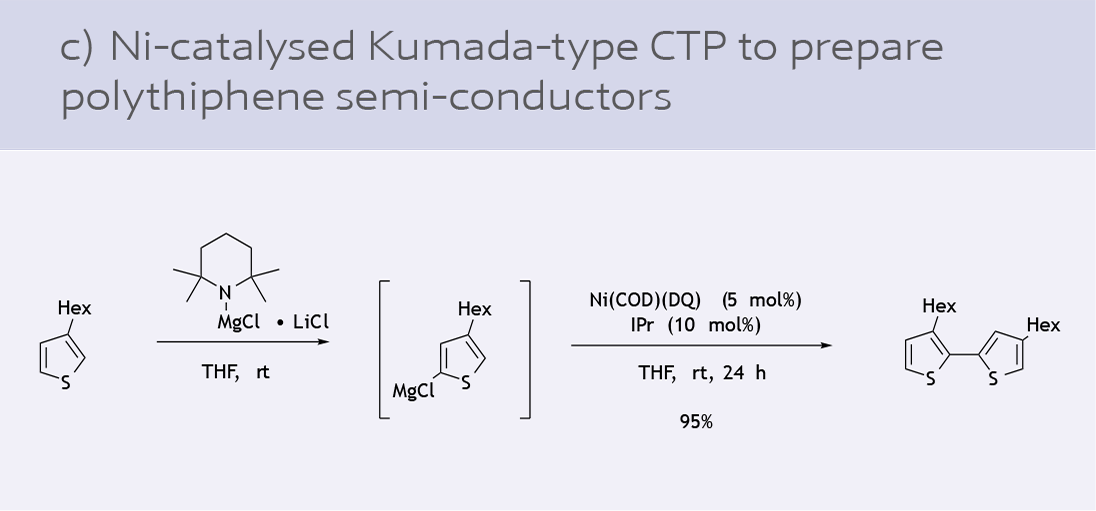
Get in touch
Send us a message to request a quote or ask our advice. We'd be happy to hear from you.
Pre-formed air-stable nickel(II) complexes
Air-stable nickel(II) complexes have also gained significant attention for a variety of novel catalysis applications such as photoredox, cross-electrophile, and electrochemical coupling reactions. In addition, nickel phosphine complexes have expanded the scope of viable electrophilic coupling partners within cross-coupling methodologies due to the inherent reactivity and selectivity differences between nickel and palladium organometallic complexes.
Over the past two decades NiCl2(PCy3)2 (Ni-114) has proven to be an effective catalyst for cross-couplings utilising aryl pivalates, tosylates, carbamates, ethers, amides, and other non-traditional electrophiles (13).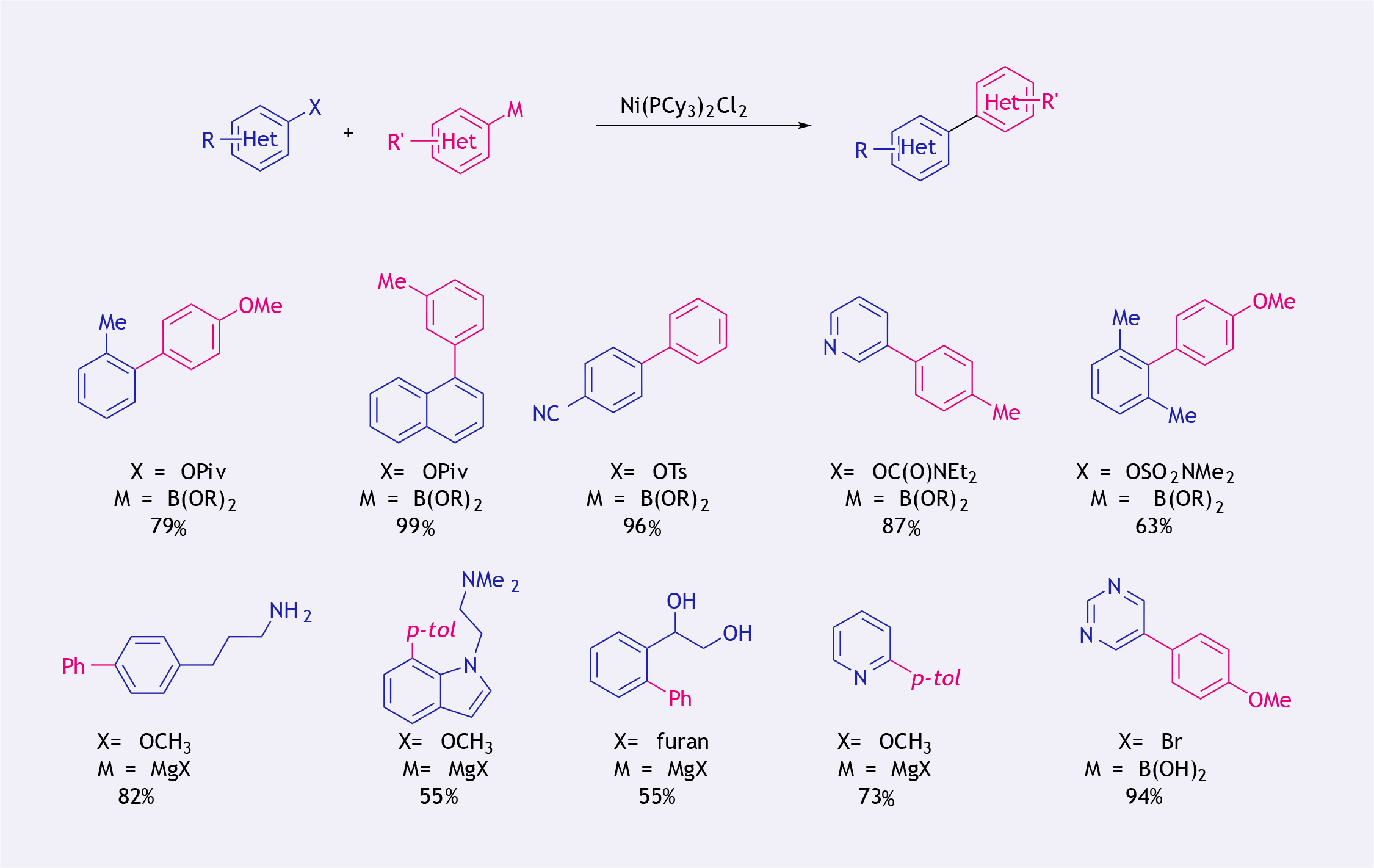
Representative substrates for Ni-catalyzed catalyst transfer-polycondensation (CTP) using Ni(dppe)CL2 and Ni(dppp)Cl2
Within the performance materials industry, Ni(dppe)Cl2 (Ni-103) and Ni(dppp)Cl2 (Ni-126) are privileged catalyst systems for preparing poly-3-hexylthiophene semiconductive polymers via a catalyst-transfer polymerisation (CTP) chain growth mechanism. The resulting polymers can be produced with excellent control over the polymer molecular weights, high regioregularities and narrow dispersity.
Advancements and looking ahead
While Ni homogeneous catalysts possess a bright future for industrial applications, several challenges associated with base metal catalysis still needs to be overcome. Many of the methodologies described above require high catalyst loadings or require expensive specialty ligands/additives. From a sustainability perspective, nickel does not have a well-established global refining loop like platinum group metals and the regulatory implications for the use of nickel catalysts in human health applications are not fully understood. Despite these challenges, base metal catalysis will continue to grow and play an important role in the future of the life science and fine chemical industries.
Ni complexes available from JM
Catalogue | Nickel complex | Mol weight | % metal | CAS number |
---|---|---|---|---|
Ni-103 | NiCl2(dppe) | 528.02 | 11.12 | 14647-23-5 |
Ni-107 | NiCl2(dppf) | 683.98 | 8.58 | 67292-34-6 |
Ni-114 | NiCl2(PCy3)2 | 690.46 | 8.50 | 19999-87-2 |
Ni-126 | NiCl2(dppp) | 542.05 | 10.83 | 15629-92-2 |
Ni-127 | NiCl2(dcypf) | 708.18 | 8.29 | 917511-89-8 |
Ni-128 | NiCl2(PPh3)2 | 654.17 | 8.97 | 14264-16-5 |
Ni-129 | Ni(COD)2 | 275.06 | 21.34 | 40759-64-6 |
Ni-131 | Ni(COD)(DQ) | 301.48 | 17.73 | 40759-64-6 |
Get in touch
Send us a message to request a quote or ask our advice. We'd be happy to hear from you.
References:
1. (a) Org. Process Res. Dev. 2023, 27, 11, 1913–1930; (b) Org. Process Res. Dev. 2021, 25, 7, 1471–1495; (c) Org. Process Res. Dev. 2022, 26, 8, 2281–2310; (d) Org. Process Res. Dev. 2022, 26, 1, 14–42; (e) Org. Process Res. Dev. 2021, 25, 8, 1802–1815, (f) Org. Process Res. Dev. 2020, 24, 6, 909–915.
2. (a) ACS Catal. 2015, 5, 2, 631–636; (b) ACS Catal. 2014, 4, 3, 999–1003; (c) Chem Rev, 2015, 115, 12091; (d) Polym. Chem., 2019,10, 2354-2369.
3. J. Am. Chem. Soc. 2023, 145, 11005-11011.
4. Org. Process. Res. Dev. 2015, 19, 1356-1359.
5. J. Am. Chem. Soc. 2024, 146, 13, 9172-9180.
6. J. Am. Chem. Soc. 2023, 145, 18366-18381.
7. J. Am. Chem. Soc. 2024, 146, 2419-2425.
8. ACS Catal. 2022, 12, 15, 8904-8910.
9. (a) Angew. Chem. Int. Ed. Engl. 2020, 7409 (b) Angew. Chem. Int. Ed. Engl. 2023, e202211794
10. J. Am. Chem. Soc. 2021 4817-4823.
11. Org. Biomol. Chem. 2024, 22, 2574-2579.
12. Org. Lett 2022, 6642-6646.
13. (a) J. Am. Chem. Soc. 2008, 130, 44, 14422-14423.; (b) J.Org. Chem. 2012, 77, 6, 2885-2892; (c) Angew. Chem. Int. Ed. 2004, 43, 2428-2432; (d) Org. Lett. 2013, 15, 3950-3953; (e) ACS Catal. 2018, 8, 10, 9131; (f) Org. Lett. 2019, 21, 4, 1226-1231.
14. Chem. Rev. 2016, 116, 4, 1950–1968; (b) Acc. Chem. Res. 2016, 49, 2822-2831; (c) ACS Macro Lett 2016, 5, 332-336.