Biocatalysis
Biocatalysis
We’re finding new ways to speed up our customers’ industrial processes using enzymes taken from nature and modified for new purposes.
14 February 2018
Enzymes are the accelerator pedals of the natural world. Made up of amino acids, they are the essential building blocks of life; biomolecules that speed up or ‘catalyse’ a dizzying range of biochemical reactions, from wound healing to digesting food.
But they can potentially do even more. With a bit of development, enzymes can also catalyse many of the industrial reactions that produce the chemicals and materials that are essential for our modern world. Enzymes, in theory, have several advantages over synthetic catalysts, as they are more selective, meaning they can produce more of the desired product and less undesired by-products. Because they are performing the same function as synthetic catalysts, we refer to these industrial enzymes as biocatalysts.
Improving on nature
For thousands of years, humans have made use of enzymes, even if until recently they did not realise they were doing so. An early example is beer making, where enzymes in yeast, a fungus, speed up the conversion of sugar into alcohol. But as scientists have come to understand more about the power and abilities of enzymes over the past 150 years, they have been applied to a range of uses, from processing food to cleaning clothes in biological washing powders.
Nevertheless, until recently, scientists were more reliant on enzymes provided by nature, which had evolved to aid their host cells rather than be useful for mankind. “Enzymes that occur in nature are ‘wild type’ and do not always do what chemists want them to do,” says Professor Manfred Reetz at the University of Marburg in Germany, one of the authorities in the field.
Reetz and other pioneering scientists, such as Frances Arnold, explored the concept of “directed evolution” or “artificial evolution”, in which natural evolution was mimicked in a laboratory setting in much shorter timescales. By randomly mutating the natural enzyme to generate a library of enzyme variants and subsequently screening them for a target reaction to find improvements, they demonstrated the potential of modifying natural enzymes to perform better as catalysts for man-made reactions.
“We started as proof of principle twenty years ago or more. At that time, it wasn’t industrially viable because the mutagenesis techniques took too long and the libraries were too big,” he says.
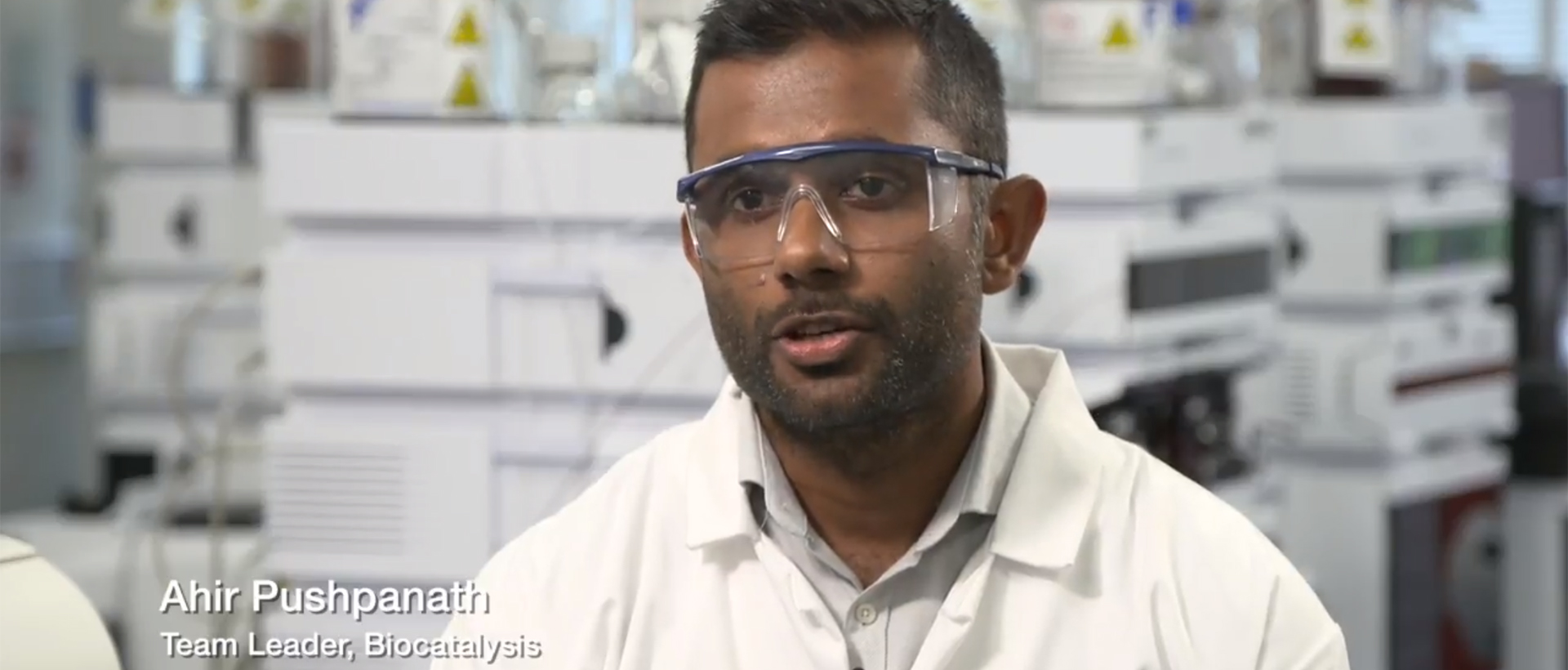
A numbers game
Directed evolution has allowed industrial biochemists to produce enhanced enzymes, but it’s slow, generally taking up to a year to transform a natural enzyme into a version with a desired ability for industrial use. This owes to the fact that every enzyme variant must be screened to see if it improves upon the original and commonly, a large number of variants need to be tested before discernible improvements are found. This can considerably slow down the process and is akin to finding a needle in a haystack; even changing five amino acids (to all the other possible natural amino acids) in the natural enzyme generates millions of enzyme variants for testing. As a result, scientists like Reetz have been trying to develop methods in which we can “screen smarter” by creating smaller libraries of variants with higher chances of finding improvements.
When we decided to complement our expertise in conventional catalysts with biocatalysts, we turned to computational modelling to overcome the “numbers problem” of directed evolution. “Computational approaches may be the future of this field,” says Ahir Pushpanath, team leaderin biocatalysis at Johnson Matthey.
“What we’re doing is building on existing research to develop unique computational methods,” explains Ahir. His team uses in-house developed software tools and huge computerised databases to try to predict the best existing natural enzyme for a desired chemical reaction. “We fish out enzymes that are most likely to have the required capacity to do the reaction,” he says. “If it already exists in nature, we don’t need to engineer it.”
Where there isn’t a suitable natural enzyme for the desired reaction, Ahir and his team move onto enzyme engineering using smart library design, rather than directed evolution. Here, they compare the DNA sequence (genetic code), the amino acid sequence and predicted 3-D structure of the encoded enzyme for thousands of enzyme variants. They use these, in addition to other computational biology tools, to identify which alterations are most probably required to improve a given enzyme. Although the method is not fool-proof, it drastically reduces the number of enzyme variants that need to be screened before finding improvements. He says: “Unlike conventional direct evolution, this process is quicker and on average we can often discover a suitable enzyme with the correct abilities within two months.”
From computer to customer
From a practical viewpoint, library construction is performed by the molecular biologist team, who specialise in techniques to mutate the DNA sequence (of the original natural enzyme) according to the design proposed by the computational team. These scientists then insert the DNA coding for the enzyme variants into fast-growing bacteria called Escherichia coli. Our E. coli are tailored to produce vast quantities of the target enzyme as part of their life cycle.
The next step involves breaking open the E. coli to extract the enzyme variants, which we test for their ability to catalyse the desired reaction. Regardless of smart library design, these can still be in the range of tens of thousands of enzyme variants, necessitating the use of the latest high throughput screening technologies to speed up the process.
Results from the testing process are subsequently fed back to the computational team to improve their predictive ability. Usually, even after a few rounds of this improvement process, we have an enzyme that can efficiently catalyse the desired reaction, which we can supply to customers in the form of a powder.
The future of biocatalysis
Already, we have developed and can supply enzymes able to catalyse a range of common industrial reactions, particularly reactions that involve adding hydrogen atoms to molecules, known as hydrogenation reactions. We are now applying our computational-based enzyme engineering techniques to some of today’s biggest industrial challenges, from pharmaceuticals and agrochemicals to flavours and fragrances. The future of biocatalysis relies on our ability to engineer natural enzymes more efficiently, both scientifically and economically. In addition, having the ability to reliably and quickly design de novo enzymes that can catalyse completely novel chemistry unseen in nature is an ambitious future goal of the field.
To do both, research into using computers to accurately predict how enzymes fold given only their amino acid sequence is crucial as unravelling the sequence-structure-function relationship of all proteins remains one of the greatest unsolved mysteries of our time. “It’s the holy grail,” says Ahir. “If you crack that relationship, you can potentially design any enzyme from scratch. The person who does it will dominate the field.”